This laser technology may prove attractive for thin metal applications
October 14, 2008
High-power diode lasers are just beginning to make an impact on welding applications. They are also physically smaller than other lasers, and their initial capital cost is not as large as it might be for traditional welding lasers because diode lasers have fewer system components.
While lasers have been used in welding for many years, this is still an active area of technological development. In particular, improvements in traditional welding lasers, together with the introduction of entirely new types of lasers, have expanded the capabilities of this technique and changed the cost characteristics of laser welding.
High-power diode lasers are just beginning to make an impact on welding applications. They are physically smaller than other lasers, and their initial capital cost is not as large as it might be for traditional welding lasers because diode lasers have fewer system components.
Laser Welding Modes
Before delving further into welding with diode lasers, it makes sense to discuss the different laser welding techniques: keyhole and conduction welding. Both of these are typically performed autogenously—that is, no filler metal is added to the joint.Keyhole, or deep-penetration, welding is probably the most common. In keyhole welding, the laser is focused to achieve a very high-power density—typically at least 1megawatt/cm2—at the workpiece. At the center of the focused beam, where the laser power density is usually highest, the metal actually vaporizes, opening up a blind hole, the keyhole, into the molten metal pool. Vapor pressure holds back the surrounding molten metal and keeps this hole open during the process. The metal vapor also radiates laser energy into the molten metal along the side of the keyhole, transferring energy through the entire depth of the keyhole and resulting in a weld with a deep aspect ratio.
The small size of the keyhole region results in a relatively small fusion zone and heat-affected zone (HAZ). Furthermore, the highly localized application of heat means that the workpiece both heats up and cools down rapidly, which can minimize grain growth in high-strength, low-alloy steels. But even though no filler material is typically used for keyhole welding, the high temperatures can vaporize volatile materials, producing a different composition in the fusion zone than in the base metal. In hardenable steels, rapid cooling generates fully martensitic fusion zones and hardened HAZs.
In contrast, if the threshold laser power required to initiate a keyhole is not reached, then only surface melting occurs. The laser energy is absorbed almost entirely at the surface, and conduction distributes the heat throughout the bulk material; conduction welding is the result. Conduction mode welds are typically shallow and have a bowl-shaped profile.
The HAZ is larger than for a keyhole weld, and the transition from the fusion zone to the base metal is smoother and more gradual.
The gentler heating cycle produced with conduction mode welding avoids the formation of martensite and generally doesn't evaporate lighter alloying metals. As a result, changes in alloy properties between the base metal and fusion zone are minimized.
Keyhole welding requires that a high threshold power be reached to start the process, resulting in a narrow process window. Keyhole mode welding is suitable for deep-penetration welds for which high aspect ratios are desirable. However, conduction welding works over a relatively large linear power range. This means that the delivered power can be adjusted until the ideal conditions for the particular application are achieved. Taken together, the combination of power control and shallow penetration makes conduction mode welding most suitable for delicate, heat-sensitive parts and thin metals.
Traditional Welding Lasers
Several different laser technologies are currently employed for welding (seeFigure 1). The specific characteristics—such as output beam and cost factors—of each laser type determine the ways in which it can be used for welding. The most commonly encountered welding laser types are CO2and solid-state (lamp-pumped or diode-pumped).
Several laser types are used in welding. Only a few make sense for keyhole mode welding.
Fiber lasers were introduced several years ago and have been adopted in some important niches. High-power diode lasers also have been available for a few years, but are still just making their entry into welding applications.
CO2lasers output well into the infrared wavelength and typically provide a high-power, well-collimated pencil beam of just a few millimeters in diameter. While the infrared light of the CO2laser is not well-absorbed by most metals, the combination of very high power and small beam diameter yields the power density necessary to initiate keyhole welding. CO2lasers are not the most electrically efficient of laser technologies, requiring more input electrical energy to be converted into useful laser light. Also, their infrared light cannot be delivered by optical fiber.
In solid-state lasers, the light from either a lamp or a series of diode lasers is focused, or pumped, into a laser rod, which then emits a small, well-collimated beam of laser light in the near infrared. This beam can be fiber-delivered. (A variant on this is the disk laser, in which the solid-state laser medium is disk-shaped instead of a rod; disk lasers are all diode-pumped.) Solid-state lasers are used mostly for conduction welding.
The basic configuration of lamp-pumped solid-state (LPSS) lasers makes them less electrically efficient than other laser technologies. The lamps also must be replaced every few months. However, this legacy technology is well-established and -understood by most in the manufacturing industry.
Diode-pumped solid-state (DPSS) lasers are less complicated and have lower consumables cost, but their initial purchase price is higher.
Fiber lasers are conceptually like DPSS lasers, but the laser rod is replaced with an optical fiber as the laser medium. Single-mode fiber lasers, which produce light that can be focused to a small spot, deliver output in the same power and wavelength range as DPSS lasers. However, they can be focused to a smaller spot to achieve the power density necessary for keyhole welding.
Multimode fiber lasers can deliver tens of kilowatts, but in a bigger spot size and with lower power density, making them suitable for conduction mode welding.
Enter Diode Lasers
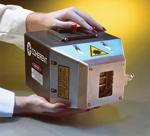
The diode laser is a small semiconductor device that uses electrical current as its energy source. Typically, higher-power diode lasers output in the near infrared, at a slightly shorter wavelength than fiber or solid-state lasers. A typical diode laser emitter might produce at most a few watts of output power. However, it is possible to fabricate numerous emitters on a single, monolithic substrate or bar with a total output as high as 100 W. These bars can, in turn, be combined in horizontal and vertical stacks to produce high-power direct diode laser systems with total output power in the multikilowatt range.
Because of the inherent optical characteristics of diode lasers, their light output spreads out rapidly once it exits the device. As a result, the light from a diode laser system cannot be focused into a small spot, precluding keyhole welding. However, it is possible to collect the output from the individual diode emitters into an optical fiber with output that then delivers the power density necessary for conduction welding.
Diode lasers offer several distinct advantages as a welding source. For example, the process of converting electrical energy into light is many times more efficient in a diode laser because fewer steps are involved in generating the laser light. There is no excitation of a gas or need to drive a pump light source, for example. The entire electricity-to-light conversion takes place directly within a semiconductor chip.
Also, because the diode lasers themselves are monolithic, miniature devices, diode laser systemstake up little room.While the semiconductor bars must be mounted on heat sinks and require external optics, the system does not have as many individual bulk components, such as laser crystals, end mirrors, and pumping devices, as other laser types do.
Welding With Diode Lasers
Diode lasers are best-used for conduction mode welding of thin metals. Because of their small size (see Figure 2), diode laser systems can be mounted directly on robot arms and moved relatively quickly. Alternatively, the ability to deliver the output through long lengths—as much as 100 feet—of optical fiber allows a high level of flexibility in terms of where the laser system is located. It also enables beam delivery into tight or hard-to-access spaces.Typical applications would include under-hood welding of components in automobile manufacturing and welding of heat-sensitive medical devices, such as pacemakers.
More important, diode lasers can be used with the same materials and alloys that are currently processed with conduction welding. Typical examples are medium- and high-carbon steels, which tend to form an undesirable martensitic fusion zone when subjected to the high temperatures and fast temperature cycling associated with keyhole welding.
The galvanized, or zinc-coated, steels commonly used in automotive applications also can be welded with diode lasers. Again, these are problematic with keyhole welding because the zinc melts rapidly, whereas the lower temperature of conduction welding results in a fusion zone that has a uniform dilution of zinc and steel with no porosity.
Diode lasers are also suitable for welding stainless steel. Typical examples are medical devices, reactors, and aerospace components. Again, this is because the lower process temperature doesn't cause removal of more volatile alloying elements from the fusion zone. Additionally, stainless steels are generally increasingly reflective at longer wavelengths, so the shorter wavelength of the diode laser results in incrementally better light absorption and, thus, higher efficiency.
The higher absorption of shorter wavelength light is even more pronounced in aluminum, which has a significant dip in its reflectivity in the near infrared. Aluminum alloys containing volatile alloying materials (such as magnesium), which are difficult to keyhole-weld, can be successfully conduction-welded with diode lasers.
Wilmer J Sánchez
V-19358601
seccion 1
Fuente: http://www.thefabricator.com/article/laserwelding/welding-with-diode-lasers
No hay comentarios:
Publicar un comentario